SUMMARY
The A-1A and titanium/potassium perchlorate (TPP) igniter compositions have been used in hand grenade fuzes for many years. However, producing or sourcing acceptable-quality A-1A has been challenging. TPP contains potassium perchlorate, which has been targeted for removal from pyrotechnics by the U.S. Department of Defense. In hand-grenade fuzes, an input charge is often used to ignite the delay composition. After a period of time, the delay composition typically ignites an output charge, causing hot gases, incandescent combustion products, and ejected titanium sparks. Conventional pyrotechnics requires a nearly-gasless input charge (A-1A) and an explosive output charge (TPP). A ternary mixture of titanium, manganese dioxide, and polytetrafluoroethylene (PTFE) can fulfill both purposes. The PTFE serves as a gas generator, lubricant, and dry binder. Pressed layers of the new titanium based igniter possess adequate mechanical strength and effectively retain delay increments that do not contain any binder. Importantly, as an input charge, the new igniter does not prematurely rupture fuze cases or eject percussion primers. Yet, as an output charge, it produces a brilliant burst of sparks similar to TPP.
INTRODUCTION
In munitions, pyrotechnic delay elements are used to time sequences of energetic events. For example, fuzes for hand grenades must provide a reliable and safe interval between when the primer is struck (the grenade is released) and subsequent initiation of the main charge. The M201A1 fuze, fitted on U.S. Army smoke grenades, contains a pyrotechnic delay element that burns for ~1.0–2.3 s. The M213 and M228 fuzes are used in the M67 and M69 fragmentation and practice grenades, respectively. These munitions require a 4.0–5.5-s delay time. The M208 fuze provides an 8–12-s delay time and is used in smoke pots, which are large canisters filled with smoke-producing pyrotechnic compositions. Other specialized pyrotechnic delay elements in munitions provide delay times of 15–20 s or longer, depending on functional requirements. A generalized configuration is shown in Figure 1. In hand-grenade fuzes, the initiator is typically a percussion primer.
![Figure 1: Cross-Sectional Representation of an Exemplary Pyrotechnic Delay Element: (1) Case, (2) Initiator, (3) Headspace, (4) Igniter Composition (Input Charge), (5) Delay Composition, and (6) Another Igniter Composition (Output Charge) (Source: U.S. Army Combat Capabilities Development Command [CCDC] Armaments Center).](/wp-content/uploads/2019/11/dsiacjournal_spring2019_tiigniter_shaw_fig1-245x393.jpg)
Figure 1: Cross-Sectional Representation of an Exemplary Pyrotechnic Delay Element: (1) Case, (2) Initiator, (3) Headspace, (4) Igniter Composition (Input Charge), (5) Delay Composition, and (6) Another Igniter Composition (Output Charge) (Source: U.S. Army Combat Capabilities Development Command [CCDC] Armaments Center).
The pyrotechnic delay formulations typically used in hand grenade fuzes use objectionable chemicals such as barium chromate, lead chromate, and potassium perchlorate. In this context, the nearly-gasless Mn/MnO2 and W/MnO2 thermitic systems have been explored as possible replacements [1, 2]. However, there are other problematic components in such fuzes. As an example, within the M201A1 fuze, the delay composition is typically ignited by a thin layer of igniter composition—the input charge. At the other end of the fuze, the delay composition ignites a second igniter—an output charge that ruptures the case and ignites the contents of the grenade attached to the fuze. The first igniter, A-1A, contains zirconium, red iron oxide, and diatomaceous earth. Due to stringent military requirements for the performance of A-1A igniter composition and the fine, powdered zirconium that it contains, manufacturers have found it challenging to produce or obtain this igniter in a form suitable for fuzes. The second igniter, the output charge, is a mixture of titanium and potassium perchlorate (TPP); it is objectionable due to the presence of the perchlorate salt.
The delay element of Figure 1 is not a vented design. The headspace is sealed by the case, the initiator, and the pyrotechnic compositions. The case and initiator are not designed to vent gases or gas pressure that may accumulate within the headspace while the input charge and delay composition burn. Gas pressure within the headspace may or may not be relieved once the output charge ignites. Whether this occurs or not depends on the porosity of the combustion products produced by the input charge and the delay composition. Nevertheless, the conventional protocol has been to use a “gasless” igniter (A-1A) as an input charge and an explosive one (TPP) as an output charge. This is partly because a gas-producing input charge could eject the initiator or prematurely rupture the case. In contrast, an output charge must produce some amount of gas to forcefully eject the hot combustion products and metal sparks required to ignite the pyrotechnic contents of a smoke grenade.
Surprisingly, mixing titanium, manganese dioxide, and PTFE can produce acceptable input and output charges for hand-grenade fuzes. The Ti/MnO2/PTFE composition produces enough gas as an output charge to forcefully eject incandescent molten oxides and titanium sparks, yet the same composition may be used as an input charge without causing the ejection of percussion primers or premature rupturing of fuze cases [3].
The Ti/MnO2/PTFE composition produces enough gas as an output charge to forcefully eject incandescent molten oxides and titanium sparks.
EXPERIMENTS
Material Properties
The materials used in this study, all fine powders, were used as received. Vendor information, material specifications, and nominal particle sizes are shown in Table 1. Figures 2 and 3 are scanning electron micrographs of the titanium powder and manganese dioxide powder, respectively. The former was distinctly coarser than the latter.
Table 1: Material Vendors, Specifications, and Nominal Particle Sizes
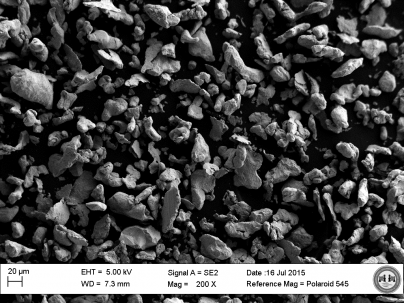
Figure 2: Scanning Electron Micrograph of Titanium Powder (×200) (Source: CCDC Armaments Center).
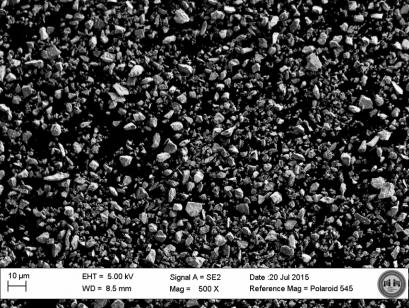
Figure 3: Scanning Electron Micrograph of MnO2 Powder (×500) (Source: CCDC Armaments Center).
Preparation of Pyrotechnic Compositions
Igniter and delay compositions for fuze assembly and sensitivity tests were prepared by combining shaking and screening steps. Shaking was performed remotely with a Scientific Industries Vortex Genie. The dry powders were combined in conductive containers and shaken for 5 min, passed through a 100 mesh screen twice, and then shaken for another 5 min.
Sensitivity Testing
Impact sensitivity tests were performed with a Bundesanstal für Materialforschung und -prüfung (BAM) 5-kg drop hammer. A Chilworth BAM friction apparatus was used for friction sensitivity testing, and a Safety Management Services (Alleghany Ballistics Laboratory) apparatus tested electrostatic discharge (ESD) sensitivity. The reported values represented the greatest energy or force resulting in nonignition for 10 (impact and friction) or 20 (ESD) successive trials.
Thermochemical Calculations
Thermodynamic equilibrium calculations were performed with FactSage 7.0 [4]. FactPS, FToxid, and a custom database that included thermodynamic data for PTFE were used. The custom database was professionally built by The Spencer Group, Inc., using available literature data [5]. All simulations assumed a constant pressure of 101.325 kPa, with the reactants initially at 298.15 K. Analyses performed in adiabatic mode (ΔH = 0) gave predicted adiabatic reaction temperatures (Tad) and the equilibrium products at those temperatures.
Fuze Assembly and Testing
Each M201A1 or M213/M228 fuze was prepared by pressing an output charge, a delay composition, and an input charge into fuze hardware with a hydraulic press at 200 MPa. The pyrotechnic compositions were loaded and pressed in one to four increments, depending on the type of fuze. A percussion primer and primer holder (if necessary) were then fitted, and the aluminum delay case or zinc fuze body was crimped to secure them.
To perform each functioning test, a fuze was fitted with a hinge pin and striker and mounted in an insulated clamp attached to a rigid assembly. A steel weight was positioned approximately 60 cm above the fuze within a plastic tube and held in place by an electromagnet. The weight was dropped by turning off the power supply to the electromagnet. The action of the weight on the striker initiated the fuze by firing the percussion primer. The signature produced by the weight striking the fuze was captured by an acoustic trigger (Kapture Group MD-1505 with transistor-transistor logic [TTL] output). The striking/initiating event caused the acoustic trigger to generate a 5-V TTL pulse used to activate an in house-developed data collection system. The audible report produced by deflagration of the output charge generated a second TTL pulse. The time difference between the two pulses was used as the fuze functioning time. The accuracy of the method was verified with a high-speed video camera (Vision Research Phantom 7.1). The delay burning time most likely accounted for much of the functioning time, as the other events were rapid.
RESULTS AND DISCUSSION
Calculated Properties
Table 2 lists some calculated properties of five different igniter compositions. The first, IC-1, is known as A-1A. The second, IC-2, is known as TPP. Compositions IC-3, IC-4, and IC-5 are experimental. Calculated adiabatic reaction temperatures and the amounts of gas products predicted to form at those temperatures are presented. Here, chemical equilibrium is assumed. For example, IC-5 is expected to produce as much as 21.90 wt-% gas upon combustion. However, the actual combustion temperatures are likely to be lower because of heat lost to the surroundings, and the actual amounts of gas produced may vary. These calculations do not consider atmospheric interactions, which are relevant in certain fuel-rich systems such as the titanium-based ones in Table 2. However, the results provide useful and quantitative indications.
Table 2: Calculated Properties of Igniter Compositionsa
Sensitivity Assessment
The sensitivities of the igniter compositions in Table 2 regarding various ignition stimuli were determined and are shown in Table 3. They suggest that compositions IC-3, IC-4, and IC 5 should generally be safer to produce and handle than A-1A or TPP. Nonetheless, appropriate precautions should always be taken when preparing or handling pyrotechnic compositions. Overall, IC-5 appears to be the least sensitive of the five compositions.
Table 3: Sensitivity Data for Igniter Compositionsa
Pyrotechnic Chemistry and Gas Production
For many years, the A-1A igniter has been used as an input charge in fuzes. It produces a negligible amount of gas upon combustion. The hot condensed-phase products formed, including molten iron, effectively ignite pyrotechnic delay compositions. However, it is unsuitable for use as an output charge because it does not produce enough gas. In contrast, TPP is explosive and produces a substantial amount of gas. Potassium chloride, volatile at pyrotechnic temperatures, is a primary constituent of the gas. The condensed-phase products include titanium oxides and excess titanium metal in the liquid state. Droplets or particles of titanium metal ejected from the combustion zone continue to burn in the air at a very high temperature. Generally, effective output charges produce an appropriate distribution of condensed-phase and gas-phase products upon combustion and the gas forcefully ejects the condensed-phase products (and excess metallic fuel, if present). While the presence of titanium in an output charge is not required, it is generally advantageous because an excess of the metal readily forms the aforementioned sparks, effectively igniting other pyrotechnic compositions.
In compositions IC-3, IC-4, and IC-5, the Ti/MnO2 thermitic system is predominant. DE is usually thought of as an inert material. However, in systems containing group 4 metals (titanium, zirconium, or hafnium), the formation of silicides is plausible. On the other hand, PTFE is undoubtedly reactive. The pyrotechnic chemistry of the Ti/MnO2 and Ti/PTFE systems may be approximated by six representative chemical equations. Equations 1–3 are more likely to occur when the mixtures contain low titanium loadings or are deficient in titanium. Equations 4–6 correspond to high titanium loadings and are more likely to occur in titanium-rich mixtures. Excess titanium can also undergo combustion in the air, as shown by equation 7.
In these equations, at the anticipated temperatures of combustion, carbon and titanium carbide (C and TiC) are in the solid state, the titanium oxides are expected to be liquids, the manganese metal likely exists as a mixture of liquid and gas, and the titanium fluorides are certainly gases. Thus, it may be understood how adding PTFE to Ti/MnO2 mixtures increases the amount of gas produced. Further, this can be achieved when titanium is present in excess, at loadings greater than about 50 wt-%. Note that the amounts of gas expected from IC-2 and IC-5 in Table 2 are quite similar.
Open-Burning Properties
Ignition tests were conducted to reveal the pyrotechnic characteristics of the titanium-based igniter compositions in Table 2. Piles of the unconsolidated compositions, each weighing 3 g, were ignited with an electrically-heated, nickel-chromium wire. Upon ignition, the piles burned rapidly, producing a bright white flash and a burst or spray of incandescent sparks. The most violent, rapid, and explosive event was produced by TPP (IC-2). The other compositions burned somewhat more slowly. In similar tests, the same compositions were consolidated into pellets weighing 1.5 g each. Igniting the pellets produced similar and analogous pyrotechnic events, although pellets of composition IC-3 could not be ignited by an electrically-heated wire. Figure 4 shows a series of images from an IC-5 pellet test where ignition was achieved with an electrically-heated nickel-chromium wire. All of the compositions, as piles or as pellets, burned rapidly in a general sense. The combustion events were complete within 1 s. Furthermore, the combustion rates are expected to increase when the compositions are confined within a metal housing. Gas-producing compositions generally burn more rapidly, or even explosively, when confined.
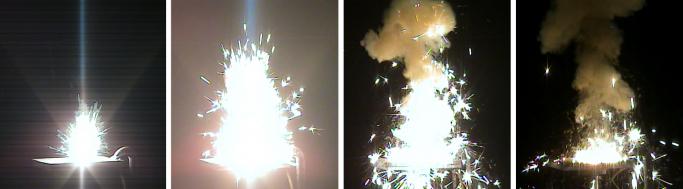
Figure 4: Open-Air Combustion of a 1.5-g Pellet of IC-5 (Source: CCDC Armaments Center).
Hand-Grenade Fuzes
Within a hand-grenade fuze, the input charge must possess mechanical integrity so that it does not disintegrate into the headspace during transportation or when the grenade is thrown. For this reason, the A-1A igniter has often been mixed and granulated with a small amount of vinyl alcohol-acetate resin. On the other hand, output charges do not necessarily require mechanical strength, especially in designs where they are sealed by the case (e.g., the M201A1 fuze). A binder is required for designs where the output charge is exposed. More importantly, binders, as reactive components or as additives, can allow the use of one formulation for both purposes.
The only composition in Table 2 that could plausibly fulfill both roles is IC-5. Here, PTFE is not only a critical reactive component but also a lubricant and dry binder. A small amount of PTFE can impart substantial mechanical strength to pressed mixtures of powdered materials. This was observed qualitatively when preparing the pellets for open-burning experiments.
The Ti/MnO2/PTFE igniter system was tested in M201A1 and M213/M228 configurations with Mn/MnO2 and W/MnO2 delay compositions. Mn/MnO2 compositions tended to burn more quickly and were suitable for the M201A1 fuze, while the slower-burning W/MnO2 system met the M213/M228burning time requirements. Delay time data for experimental M213/M228 fuzes have been reported [2]. Successful results were also obtained in the M201A1 configuration and will be reported at a later date. Figure 5 illustrates the M201A1 fuze configuration.
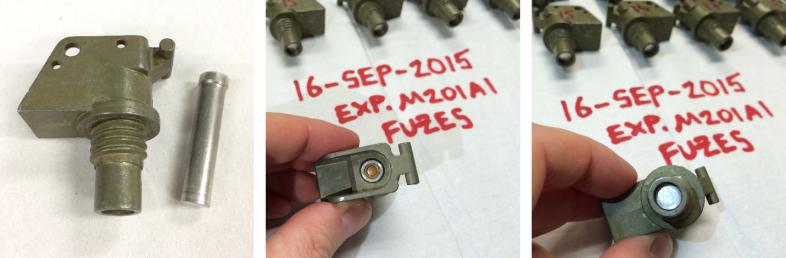
Figure 5: An M201A1 Fuze Body and Delay Case (Left), Top of an Assembled Fuze Showing the Primer (Middle), and Bottom of an Assembled Fuze Showing the Closed End of the Delay Case (Right) (Source: CCDC Armaments Center).
One benefit of using IC-5 as an input and output charge is that the delay composition need not contain any binder.
One benefit of using IC-5 as an input and output charge is that the delay composition need not contain any binder. The igniter layers are mechanically sound and effectively secure the delay increments. In hand-grenade fuzes, an effective charge weight of IC-5 is ~60–70 mg. This ensures reliable ignition of the delay column and that a robust burst of sparks is produced after the specified interval. Critically, as an input charge, the Ti/MnO2/PTFE composition does not cause primer ejection or premature case rupture, despite the fact that it produces a notable amount of gas upon combustion.
Output Charge Characterization
One of the fuze output charge events was captured with a high-speed video camera operating at 250 frames per second (Figure 6). In this figure, an M201A1 fuze is held and secured by a clamp. Above the fuze, a steel weight rests within the transparent plastic tube that it was dropped through to initiate the test. The output charge creates a burst of incandescent reaction products and titanium sparks. The initial burst occurred rapidly, within 4 ms. In this particular example, the ejection and combustion of material from the fuze was mostly complete within ~60 ms. These bursting events are typically characterized by a bright sparky flash and an audible report.
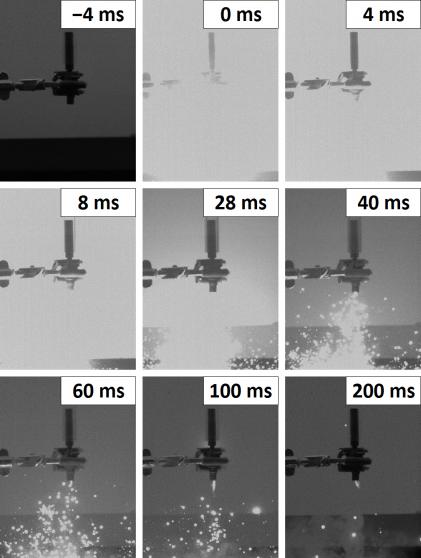
Figure 6: A Sequence of Images Showing Output Charge Combustion (Source: CCDC Armaments Center).
CONCLUSIONS
The Ti/MnO2/PTFE igniter may be used as an input and output charge in hand-grenade fuzes. Conventional igniter compositions A-1A and TPP are not required. As an input charge, the new igniter does not prematurely rupture fuze cases or eject percussion primers. Yet, as an output charge, it produces a sparky burst similar to TPP. It may be prepared as a dry mixture, without any solvent-based processing steps. Thin, consolidated layers possess adequate mechanical strength. As a result, the delay compositions do not require any binder, as the igniter layers securely retain the delay increments.
Acknowledgments:
Funding for this work was provided by the Strategic Environmental Research and Development Program, project number WP-2518, “Environmentally Sustainable Gasless Delay Compositions for Fuzes.” Robert A. Gilbert, Jr. (U.S. Army CCDC Armaments Center) is thanked for designing the tool sets for fuze assembly, and Rajendra K. Sadangi (U.S. Army CCDC Armaments Center) is thanked for acquiring the scanning electron micrographs.
References:
- Miklaszewski, E. J., A. P. Shaw, J. C. Poret, S. F. Son, and L. J. Groven. “Performance and Aging of Mn/MnO2 as an Environmentally Friendly Energetic Time Delay Composition.” ACS Sustainable Chem. Eng., vol. 2, pp. 1312–1317, 2014.
- Koenig, J. T., A. P. Shaw, J. C. Poret, W. S. Eck, and L. J. Groven. Performance of W/MnO2 as an Environmentally Friendly Energetic Time Delay Composition. ACS Sustain-able Chem. Eng., vol. 5, pp. 9477–9484, 2017.
- Shaw, A. P., J. C. Poret, L. J. Groven, J. Koenig, and J. S. Brusnahan. “Pyrotechnic Delay Element Device.” U.S. Patent Application 15/891,557, 8 February 2018.
- Bale, C. W., A. D. Pelton, W. T. Thompson, G. Eriksson, K. Hack, P. Chartrand, S. Decterov, I. H. Jung, J. Melancon, and S. Petersen. FactSage, version 7.0; CRCT ThermFact Inc. and GTT-Technologies, 2015, http://www.factsage.com, accessed February 2018.
- The Spencer Group, Inc. FactSage Thermochemical Software and Consulting, http://www.spencergroupintl.com, accessed February 2018.
- Rose, J. E. “Properties of Energetic Materials Used in Ordnance Devices.” Special Publication IHSP 89-288, Naval Ordnance Station, Indian Head, MD, 1990.