an email newsletter released every month highlighting the latest articles, events, technical inquires, and voices from the community
Barium Titanate Transducers
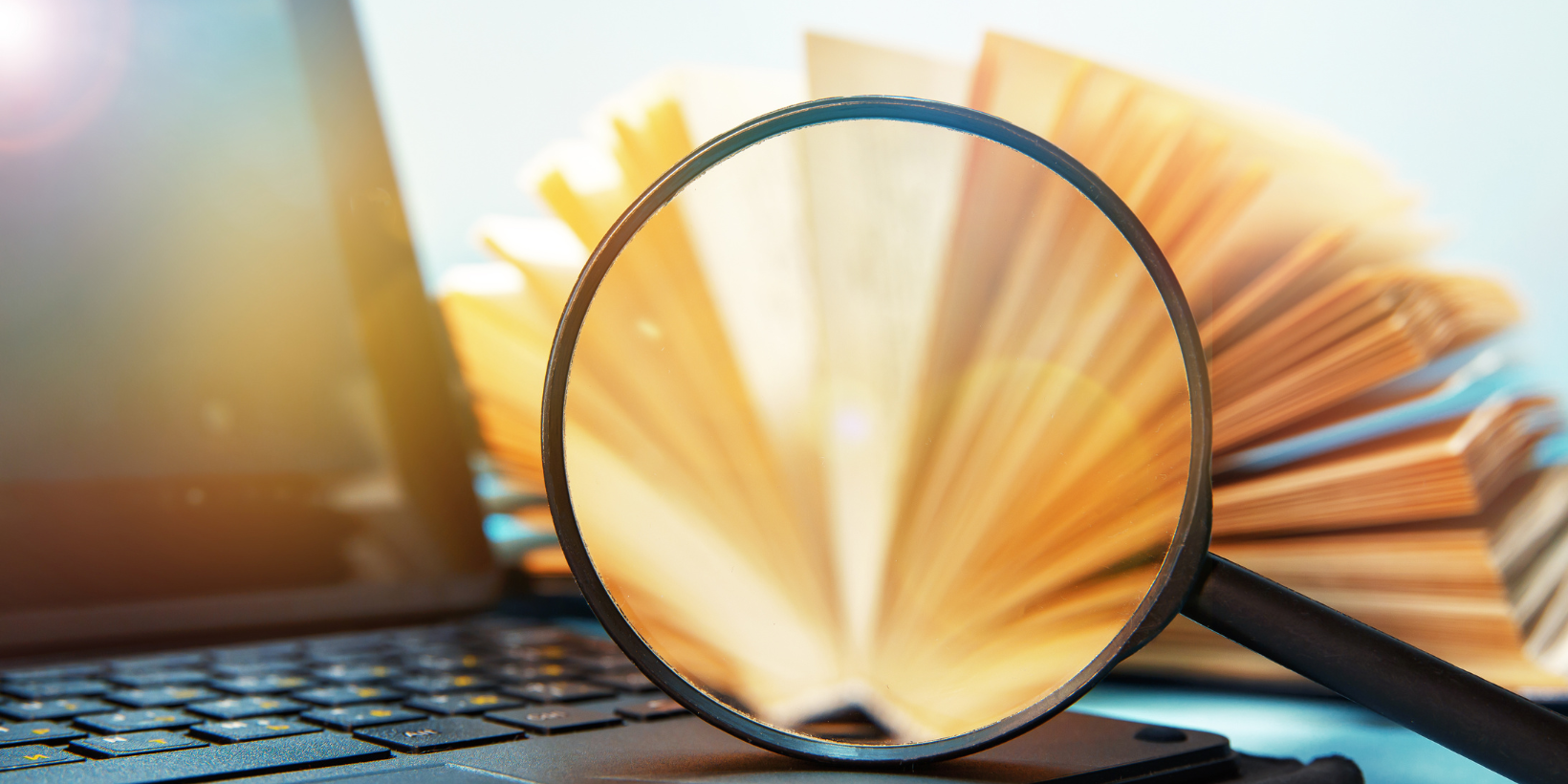
Posted on May 31, 2019 | Completed on May 31, 2019 | By: Mahesh Rao, Ph.D.
What U.S. Department of Defense (DoD) programs use barium titanate (BT) transducers, and what is the DoD demand for those transducers?
The Defense Systems Information Analysis Center (DSIAC) received a technical inquiry requesting information on barium titanate transducers and, more specifically, their use in U.S. Department of Defense organizations or their potential demand by such organizations. A DSIAC subject matter expert investigated open sources for relevant information and related organizations. This report includes a summary of barium titanate transducer technology and their applications, as well as manufacturers and potentially interested user organizations.
1.0 Introduction
Transduction refers to the conversion of energy from one form to another. In general, ceramic transducers convert electrical energy to mechanical energy and/or vice versa. This conversion is achieved by the inclusion of active piezoceramic components in the transducer, and it can take place in a variety of mediums (e.g., air, liquid, etc.) using a variety of transducer designs.
The piezo transducer’s ability to convert mechanical energy to electrical energy (and vice versa) enables the generation and detection of sound waves. Transducers that generate audible-range sound waves can be used as speakers, buzzers, alarms, etc. Transducers that generate ultrasonic-range sound waves (i.e., above 20 kHz) can be used to measure distance in various mediums, determine flow rates in fluids, monitor fluid levels in vessels, atomize liquids, perform medical imaging, weld plastics or metals, and monitor structural health.
The industrial and scientific communities have expressed a real need for pressure, acoustic, and vibration sensing at elevated temperatures. This review compares the various commercial methods and materials for acoustic transduction and identifies their advantages and limitations. Techniques and devices include simple piezoelectric sensors, accelerometers, strain gauges, proximity sensors, fiber optics, and buffer rods. Sensors with operating temperatures in excess of 650 °C are readily available from commercial sources. Of the mechanisms investigated, the piezoelectric approach offers several advantages, including design cost and simplicity. Therefore, most of this review concentrates on piezoelectric materials that are already available commercially and presently under development. The new materials include perovskite layer-structure ferroelectric ceramics, which have the highest known Curie temperatures, which are reported to be piezo-active at 1150 °C [1, 2].
BT is an important perovskite oxide with structure ABO3. BT has applications in electro-optic devices, energy-storing devices such as photovoltaic cells, thermistors, multiceramic capacitors, Dynamic Random Access Memory, etc. BT is a nontoxic and environmentally friendly ceramic with high dielectric and piezoelectric properties, so it can be used as the substitute for lead zirconate titanate (PZT) and lead titanate (PbTiO3).
Ceramic materials such as BT are characterized as being ferroelectric because the crystal domains of the material may be polarized through the application of a large electric field, and a residual polarization will remain in the material when the field is removed. These materials, when polarized, exhibit piezoelectric properties. However, materials such as BT have relatively low Curie temperatures at which they become depolarized and lose their piezoelectric properties. The Curie temperature of BT varies from 120 to 130 °C. These materials are heated when an alternating field is applied, which produces the ultrasonic energy when they are used as a transducer; therefore, the maximum power output of ceramic transducers is limited by the Curie temperature. Thus, it is desirable to use ceramic materials having high Curie temperatures in ultrasonic transducers [3, 4].
Some of the early works at the Titanium Alloy Manufacturing Company revealed that certain titanates, BT in particular, exhibited anomalous piezoelectric effects. Further studies at the Laboratory for Insulation Research at the Massachusetts Institute of Technology (MIT) established BT as a new class of ferroelectric material. The polarized polycrystalline BT showed certain analogies to ferromagnetic materials and exhibited properties which usually had been associated with single piezoelectric crystals. Until this time, the application of high power to ultrasonic transducers of the piezoelectric type had been limited by the delicate nature of the piezoelectric material and by the high voltages required. Quartz, for instance, is a stable material, but it has a very high impedance and is very expensive and difficult to produce in quantity. In comparison, Rochelle Salt has a low impedance, a low Q (qualitative factor representative of oscillation dampening in a circuit), but is relatively unstable and has definite temperature and humidity limitations. Thus, BT seemed to present a partial solution to these problems. It is relatively stable below 80 °C and has a low impedance, it is unaffected by humidity, and the basic material is relatively inexpensive and available in a great quantity [5].
To understand and further explore the piezoelectric properties, particularly from the crystallography standpoint, research began on phase transition behavior and temperature-dependent dielectric properties, as well as composition-dependent ferroelectric properties. This research included the substrate-based crystallization response of BT that adopted a hexagonal structure under substrate-free conditions as opposed to the observed tetragonal phase under substrate-supported conditions. However, in contrast to the known hexagonal BT, the substrate-free crystallized films demonstrated a small but measurable pyroelectric effect. It did not exhibit phase transitions in the 25–423 °K temperature range [6−9].
2.0 Barium Titanate Applications
A keyword search for “Barium Titanate” within the Ceramic Industry magazine website [10] revealed information about following companies that manufacture ceramic materials:
- Ferro Corp.
- Address: 6060 Parkland Blvd., Ste. 250, Mayfield Heights, OH 44124, United States.
- Website: http://www.ferro.com [11].
- Machined Ceramics Inc.
- Address: Industrial Park 629 N. Graham St., Bowling Green, KY 42101.
- Website: http://www.machinedceramics.com [12].
- CerPo Tech.
- Address: Kvenildmyra 6, Tiller, 7093, Norway.
- Website: http://www.cerpotech.com [13].
- BassTech International.
- Address: 400 Kelby St., Fort Lee, NJ 07024.
- Website: http://www.basstechintl.com [14].
- The Jet Pulverizer Company, Inc.
- Address: 1255 N. Church St., Moorestown, NJ 08057-1166.
- Website: http://www.jetpulverizer.com [15].
- Reade Advanced Materials.
- Address: P. O. Drawer 15039, Riverside, RI 02915.
- Website: https://www.reade.com [16].
- Ceramic Color & Chemical Manufacturing Company, Inc.
- Address: P. O. Box 297, New Brighton, PA 15066.
- Website: http://www.ceramiccolor.com/ [17].
- Precision Ferrites & Ceramics (PFC).
- Address: 5432 Production Drive, Huntington Beach, CA 92649.
- Website: https://semiceramic.com [18].
In addition to a host of other ceramic materials, BT was listed on the PFC website (https://semiceramic.com) in relation to development and manufacturing contracts for DoD and Department of Energy programs that involve radar systems, test and equipment components, electronic surveillance, infrared (IR) systems, and precision electromechanical devices. Additionally, other security, defense, and military applications supplied by PFC include the following [18]:
- Airport security systems
- Body armor
- Chemical agent monitors
- Decoys
- Dipping sonar
- Electronic countermeasures
- Fingerprint scanners or sensors
- Gyroscopes
- Image intensifiers (e.g., night vision)
- IR detectors
- Laser range finders
- Proximity switches
- Scanners
- Sonar buoys
- Submarine and surface sonar
- Torpedo guidance
- Towed arrays
- Vehicle armor
The report “Ad Hoc Subcommittee Report on Piezoceramics – Revision of DOD-STD-1376A (SH)” [19] provides the collective thoughts of government agencies, laboratories, industrial manufacturers, and universities that reviewed and critiqued existing standards. It also provides information on suggested areas for clarification and improvement of piezoelectric ceramic material (including BT, type IV) and measurements for sonar transducers, in addition to the inclusion of other materials, expanding user guidelines, and hydrophone and projector specifications combined for simplicity. The report also provides a usable document to both the Navy and contractors to more accurately specify piezoceramic material. The Program Manager (NRL- Underwater Sound Reference Detachment [USRD] Code 5977) for the Sonar Transducer Reliability Program (STRIP) and the sponsor (Naval Sea Systems Command [NAVSEA] 63X5) have approved the specification for distribution to Navy agencies and support contractors [19].
In the report, the following members of the subcommittee also provided some reference to the stakeholder interests about potential users of the BT ceramics [19], including the following organizations:
- NAVSEA.
- Naval Underwater Systems Center (New London, CT and Ft. Lauderdale, FL).
- NRL and ONR (Arlington, VA).
- NRL-USRD (Orlando, FL).
- Naval Ocean Systems Center (Norfolk, VA).
- AT&T Technology/Bell Labs.
- EDO Corporation, Western Division.
- Channel Products, Inc.
- Vernitron Piezoelectric Division.
Additional affiliations were solicited and included in the report, including the following [19]:
- Naval Coastal Systems Center (Panama City, FL).
- Naval Undersea Warfare Engineering Station (Keyport, WA).
- Naval Weapons Support Center (Crane, IN).
- Transducer Repair Facility, Mare Island Naval Shipyard (Vallejo, CA).
- Battelle Instrument (Richland, WA).
- Bendix Corporation (Sylmar, CA).
- Dyna-Empire, Inc. (Garden City, NY).
- General Electric (Syracuse, NY).
- Gould, Inc. (Cleveland, OH).
- General Instrument Co. (Westwood, MA).
- International Transducer Corporation (Goleta, CA).
- Raytheon Co., Submarine Signal Division (Portsmouth, RI).
- Sanders Associates (Hudson, NH).
- Magnavox Co. (Ft. Wayne, IN).
- Honeywell, Inc. (Everett, WA).
- Ametek-Straza (El Cajon, CA).
- Hughes Aircraft Co. (El Segundo, CA).
- Hazeltine, Inc. (Braintree, MA).
- Sparton Corporation (Jackson, MI).
- Westinghouse Electric Co. (Annapolis, MD).
- Texas Research Institute, Inc. (Austin, TX).
- Material Research Laboratory, Penn State University (University Park, PA).
- Applied Research Laboratory (Austin, TX).
The subcommittee also looked toward future improvements in piezo technology. There are many areas that need continuing Navy sponsorship and government or industry investigation; most of these are in the areas of measurement and test techniques, such as the following [19]:
- Measurement technique for d33 optimization.
- Measurements of other shapes (other than discs and rings).
- Strength of materials and test methods (for shock hardening).
- Measurement of piezo properties under multiple constraints (e.g., voltage, temperature, pressure, etc.).
- Corona measurements.
- Mechanical stress dependency (i.e., the influence of static stress).
- Temperature (i.e., the influence of heat stabilization after polarization).
- Additional compositions.
- Optimization of adhesion (e.g., tape tests, etc.).
Additionally, the NAVSEA Enterprise Long-Range Acquisition Forecast for 2019−2021 is shown in the Appendix and includes HLF-1 low-frequency transducers ($5M−$50M; N66604-13-D-1222) and ceramic stacks ($5M−$50M; GXP-21-3362) [20].
The Acoustic Transduction Materials and Devices Program (Division 332) within the ONR supports basic and applied research on materials and device technologies that primarily focus on Navy sonar and developing innovative systems that generate, detect, and suppress undersea sound for navigation, threat reduction, weapons guidance, and communication [21, 22].
References
[1] Turner, R. C., P. A. Fuierer, R. E. Newnham, and T. R. Shrout. “Materials for High Temperature Acoustic and Vibration Sensors: A Review.” Applied Acoustics, vol. 41, no. 4, pp. 299−324, 1994.
[2] Ertug, B. “The Overview of the Electrical Properties of Barium Titanate.” American Journal of Engineering Research, 2013.
[3] Hueter, T. F., and E. Dozois. “The Frequency Response of Barium Titanate Transducers.” The Journal of the Acoustical Society of America, vol. 24, no. 1, https://asa.scitation.org/doi/pdf/10.1121/1.1906857?class=pdf, 1952.
[4] McMahon, G. W. “Performance of Open Ferroelectric Ceramic Cylinders in Underwater Transducers.” The Journal of the Acoustical Society of America, vol. 36, no. 3, https://doi.org/10.1121/1.1918994, 1964.
[5] Conable, J. H. “Barium Titanate Sonar Transducers.” U.S. Naval Postgraduate School, Monterey, CA, 1956.
[6] Cady, W. Piezoelectricity: An Introduction to the Theory and Applications of Electromechanical Phenomena in Crystals. Vol. 1, New York, NY: Dover Publications, 1964.
[7] Stavitski, N., V. Lyahovitskaya, J. Nair, I. Zon, R. Popovitz-Biro, E. Wachtel, Y. Feldman, and I. Lubomirsky. “Substrate-Free Crystallization of Distorted Hexagonal Barium Titanate Thin Films.” Applied Physics Letters, vol. 81, no. 22, pp. 4177−4179, October 2002.
[8] Kim, I., Y. Avrahami, H. L. Tuller, Y-B. Park, M. J. Dicken, and H. A. Atwater. “Study of Orientation Effect on Nanoscale Polarization in BaTiO3 Thin Films Using Piezoresponse Force Microscopy.” Applied Physics Letters, vol. 86, no. 19, March 2005.
[9] Ebralidze, I., V. Lyahovitskaya, I. Zon, E. Wachtel, and I. Lubomirsky. “Anomalous Pre-Nucleation Volume Expansion of Amorphous BaTiO3.” Journal of Materials Chemistry, vol. 15, no. 39, pp. 4258−4261, 23 August 2005.
[10] Ceramic Industry. https://www.ceramicindustry.com/, accessed May 2019.
[11] Ferro Corporation. https://www.ferro.com/, accessed May 2019.
[12] Machined Ceramics Inc. http://www.machinedceramics.com, accessed May 2019.
[13] CerPoTech. http://www.cerpotech.com, accessed May 2019.
[14] Bass Tech International. http://www.basstechintl.com, accessed May 2019.
[15] The Jet Pulverizer Company, Inc. http://www.jetpulverizer.com, accessed May 2019.
[16] Reade Advanced Materials. https://www.reade.com/, accessed May 2019.
[17] Ceramic Color & Chemical Manufacturing Company, Inc. http://www.ceramiccolor.com/, accessed May 2019.
[18] Precision Ferrites & Ceramics. http://www.semiceramic.com/, accessed May 2019.
[19] Tims, A. C. “Ad Hoc Subcommittee Report on Piezoceramics – Revision of DOD STD-1376A (SH).” Naval Research Laboratory (NRL) Underwater Sound Reference Detachment, NRL Memorandum Report no. 5687, https://apps.dtic.mil/dtic/tr/fulltext/u2/a166616.pdf, 1 April 1986.
[20] Naval Sea Systems Command, “Small Business Forum – LRAF Enterprise.” https://www.navsea.navy.mil/Portals/103/Documents/Small_Business_Forum/LRAF_Enterprise.04302019xlsx%20(002).pdf, 2019.
[21] NRL. Personal communication with remote sensing scientist, 23 May 2019.
[22] Office of Naval Research. “Acoustic Transduction Materials and Devices.” https://www.onr.navy.mil/en/Science-Technology/Departments/Code-33/All-Programs/332-naval-materials/acoustic-transduction-materials-and-devices, accessed May 2019.
Appendix: NAVSEA Long Range Acquisition Forecast [20]
Want to find out more about this topic?
Request a FREE Technical Inquiry!